Aside from the odd molecule here and there, up until now we have focused pretty much on atoms (and nuclei) in isolation. A quick glance around you, though, reveals that this is really a small part of the picture. Your house is filled with solid objects, from in your hands to the wires in your walls to the ice cubes chilling in your refrigerator. We’d like now to introduce you to what quantum physics has to say about solids, and then to see how the characteristics of quantum solids can make our lives better.
The structure of solid matter and the arrangement of atoms in the solids depend upon the number and arrangements of electrons in each atom or, in other words, upon the electronic properties of the composite atoms. Although most solids around us are macroscopic in scale, all of their physical properties are due to the motion of electrons within them. Thus solid matter and all of its properties is explained—and controlled—by quantum physics.
Solids are generally divided between conductors (like the copper in your household wiring) and insulators (like the paper in this book). The difference between these is the ability to conduct electricity. If you attach a battery to a copper circuit, an electric current will flow. Insulators, on the other hand, do not conduct electricity. There is also an in-between category of materials that conducts electricity only some of the time. To this category physicists have assigned the rather unimaginative name semiconductors. You shouldn’t be surprised to learn that the difference between these types of solids is also explained by quantum physics.
DEFINITION
A conductor is a form of matter that conducts electricity when an electric field, or voltage, is applied to it.
An insulator is a form of matter that does not conduct electricity.
A semiconductor is a form of matter that can conduct electricity at elevated temperatures.
To see why, first consider two atoms separated far apart. Let’s pick on sodium, an atom with 1 electron in its outer valence shell (designated 3s) and the other 10 tucked nicely into the shells closer to the nucleus. When well separated, each atom has its own discrete, but identical, set of energy levels.
If you were to bring two atoms near to one another something unusual begins to happen. The wave functions of all the electrons begin to overlap, and the energy levels would split into two! This happens because you now have a two-atom system, and energy levels will be slightly different depending on whether the corresponding electrons have the same spin or if they have opposite spin. Your two-atom system now has a discrete set of little energy level pairs.
Solids of course have more than just two atoms. In fact, your typical hunk of stuff has about 1023 of them. What happens, then when you bring so many atoms into close proximity? It turns out that the individual energy levels will blur into a broad band due to all the wave function overlaps and spin-spin interactions.
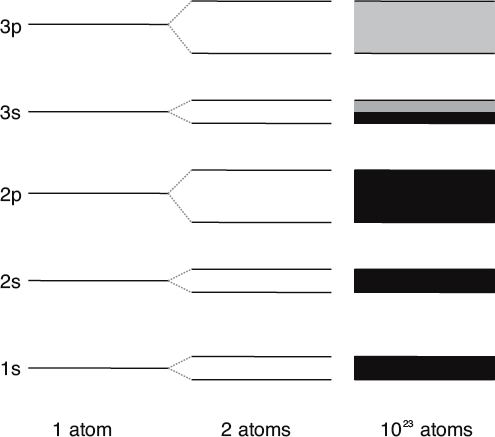
While an isolated electron has a set of single energy levels, the energy levels of a two-atom system will come in pairs. For a solid, which has more than 1023 atoms, the energy levels take the form of energy bands. For sodium, the 3s band is only halfway filled.
There is more to the story. If we return to our sodium atom, it turns out that all the electrons from the nicely filled inner states (1s, 2s, and 2p) will lead to nicely filled energy bands. But what about the band corresponding to the lone valence electrons in the 3s shell?
Recall that the valence shell for this electron can accommodate two electrons, even though sodium has just has just one there. In other words, the outermost shell of an isolated sodium atom is half full. In the solid case, this implies that the outermost band is also only half full. And, since it was formed by the atoms’ valence electrons, this band is called the valence band.
We know that a sodium atom can be excited into excited states, which means there are higher energy electron shells that are only occupied when the atom has been excited. In the solid picture, this means there are also higher lying energy bands, and these too can be occupied if the atom becomes excited. And, the band that sits nearest to the valence band is called the conduction band for reasons we’ll soon explain.
DEFINITION
The valence band is the highest occupied energy band of a quantum solid. It may be totally or only partially filed.
The conduction band is the first energy band above the valence band. Electrons promoted to the conduction band can flow in the form of electricity.
The band gap is the difference in energy between the top of the valence band and the bottom of the conduction band.
The occupancy and the proximity of these two bands is what distinguishes conductors from insulators and semiconductors. Conductors (like sodium) have partially filled valence bands. If an electric field is applied across a conductor (say, by wiring up a battery), the electrons can move and occupy one of the numerous unoccupied energy states nearby. This movement of electrons forms an electric current, better known as electricity.
By contrast, the valence bands of insulators and semiconductors are completely filled. For insulators, the energy separating the valance and conduction bands (called the band gap) is much larger than typical thermal energies. For semiconductors, however, the band gap is approximately equal to typical thermal energies. This means that if you add a little bit of heat to the system, electrons near the top of the valence band can actually be thermally excited into the conduction band, where they can flow freely. The electrons in insulators, on the other hand, are more-or-less confined to the valence band. There are no available energy states to which electrons can be promoted to take part in electrical conduction.
The electrical properties of conductors and insulators are important, though not terribly interesting. Physicists have manipulated the unique properties of semiconductors, though, to make lots of clever devices. Most notably, transistors—small electronic devices at the heart of every modern integrated circuit—are based largely on the on-again, off-again nature of semiconductors. Silicon is among the most common semiconductors, hence the origin of the name “Silicon Valley.”
Leave a Reply