Imagine you visit an old Civil War fort and observe the ceremonial firing of its cannons, and consider this question: if one were to stretch a large sheet of tissue paper across the cannon’s path, what are the chances a fired cannon ball would bounce off the paper rather than simply tear through? Impossible, right? In fact, it was the observation of something nearly so improbable that ultimately blew holes through Thomson’s plum pudding model. And much to Thomson’s dismay, this remarkable discovery was made by one of his own students, Ernest Rutherford.
It happened in 1911, when Rutherford and his own students, Hans Geiger (of Geiger counter fame) and Ernst Marsden, were conducting a series of experiments using alpha particles. Specifically, they were exploring what happened when a very thin gold foil was bombarded by an alpha-particle beam.
QUANTUM QUOTE
It was quite the most incredible event that has ever happened to me in my life. It was almost as incredible as if you fired a 15-inch shell at a piece of tissue paper and it came back to hit you.
—Rutherford’s reflection on the famous gold foil experiment.
The gold foil was so thin that the alpha particles would normally pass right through and emerge with only a very slight reduction in speed. In addition, the overall diameter of the alpha particle beam would broaden very slightly after traversing the foil. Both of these observations were consistent with Thomson’s model of the atom, since the positively charged alpha particles would undergo a series of small deflections (via electromagnetic interactions with the positive and negative components of the foil) as they sailed through.
To Geiger and Marsden’s astonishment, however, they occasionally detected an alpha particle that was scattered off to the side. Even more astonishingly, they even detected a few that were scattered backward from the foil. A simple application of Newton’s laws and Maxwell’s equations indicate that this would only be possible if all of the atom’s positive charge were concentrated in one compact region, rather than thinly distributed throughout its full volume as Thomson predicted. Rutherford named this dense, central, and positively charged region the nucleus of the atom.
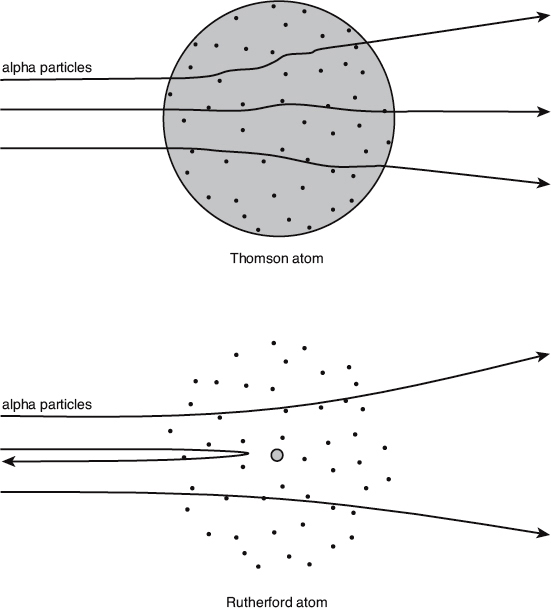
The predicted effect of alpha-particle scattering differs when comparing the Thomson and the Rutherford atoms.
QUANTUM LEAP
In addition to discovering the nucleus, Rutherford was also the first to correctly interpret the physical basis of radioactivity, for which he won the 1908 Nobel Prize. Since this phenomenon dealt with the conversion of one element to another, he was awarded the Nobel Prize in chemistry. This displeased him immensely, especially given his view that “all science is either physics or stamp collecting.”
By applying the classical physics of Newton and Maxwell, Rutherford would estimate the size of the nucleus to be approximately 1 × 10-14 m, or about 10,000 times smaller than the atom as a whole. This implied that the atom is composed of mostly empty space, and that its electrons occupy the large void surrounding a small, central nucleus. To put this into perspective, a Rutherford atom the size of Madison Square Garden would have a nucleus about the size of a marble located at center ice, while the electrons would occupy the vast space spanning all the way to the cheap seats.
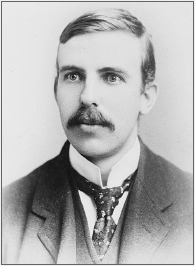
ERNEST RUTHERFORD
Ernest Rutherford was a British physicist with an unusual accent. Born in New Zealand to a Scottish farmer, he eventually traveled to England on a scholarship to the University of Cambridge. Here, his scientific career began under the great J. J. Thomson. He went on to become the father of the nuclear atom.
He was famous for clear thinking infused with simple and rugged notions, which allowed him to disentangle complex observations and draw powerful conclusions. He was equally enthused by the lofty as the mundane aspects of science, and spent many hours in the laboratory painstakingly counting the traces of alpha particles. He nevertheless made time for a second passion—literature—and is known to have once argued with the Archbishop of Canterbury over who was the more widely read.
Rutherford embodied the many successes of late nineteenth century physics. During this eventful period, in the words of C. P. Snow, “the tone of science was the tone of Rutherford: magniloquently boastful, creatively confident, generous, argumentative, lavish, and full of hope.” He truly savored his accolades, and eventually acquired such distinguished titles as Baron, Lord, and “the Crocodile.”
If you remember that oppositely charged particles attract one another, then you will immediately object to this idea. If the nucleus has a positive charge, after all, and the electrons are negatively charged, then why aren’t they simply pulled into the nucleus, thus collapsing the atom?
To answer this question, Rutherford postulated that the electrons travel in orbits about the nucleus, much like the planets in our solar system travel in distant orbits around the sun. The electron’s motion allows it to maintain a stable distance from the nucleus, such that the atom as a whole is 10,000 times larger than the central nucleus.
DEFINITION
The nucleus is the dense, central region of an atom, which comprises all of its positive charge and practically all of its mass.
A proton is a small, positively charged particle that is located within an atomic nucleus; the number of protons determines the atom’s identity.
An electron is a small, negatively charged particle that occupies the otherwise empty space around an atomic nucleus.
A neutron is an electrically neutral particle with about the same mass as a proton located within an atomic nucleus.
As time went on, Rutherford began to study the effects of alpha particle collisions with lighter and lighter atoms (as compared with gold, a relatively heavy atom). In this way, he was able to deduce that the nucleus was formed from a number of positively charged particles, which he named protons. He also determined that the magnitude of a proton’s positive charge precisely matches the magnitude of an electron’s negative charge. Since the atom is a neutral body, protons and electrons must come in pairs. Finally, he concluded, the number of protons in an atom’s nucleus determines its unique identity. Gold atoms, for example, have 79 protons, while helium atoms have 2.
Eventually, we would learn that the atomic nucleus was itself composed of protons plus one other type of particle, called the neutron. The neutron has no charge and about the same mass as the proton (that is, about 2,000 times the mass of the electron). It has the important ability to bind together all those positively charged protons that are otherwise constantly repelling one another. Protons and neutrons are bound extremely tightly in the nucleus, which is why it took a bit longer to sort all that out. There will be lots more on the “glue” that holds a nucleus together.
Leave a Reply