You may not believe it the next time you come down with a cold, but viruses are small. In fact, at tens to hundreds of nanometers, they belong to the quantum realm. As a result, they typically push the limits of electron microscopes. That explains why only a small fraction of the large variety of their genomic structures is known, and thus why the treatment of viral diseases is challenging.
Nevertheless, trailblazing research is using quantum physics to unlock the secrets of these very little beasties. This is achieved by using intense photon sources called synchrotrons. These accelerator-based photon sources use magnetic fields to accelerate electrons so that they emit intense beams of electromagnetic radiation—of much higher frequency than your typical laser because it is in the X-ray regime.
Scientists are using synchrotron radiation sources to examine biological structures in two different ways. They can either measure the emission of electrons or ions following photon absorption, or the specimen can scatter the incoming photons to produce diffraction images. The latter type of image is the basis for crystallography. These two methods provide complementary approaches to the study of living organisms from large structures down to the cell level.
Crystallography is the primary methodology used to determine three-dimensional structures. Many biological systems are difficult or impossible to crystallize, however, and consequently only a few membrane protein structures are known. In addition, damage to the specimen while undergoing photon bombardment can degrade the images.
For starters, these are up to one billion times brighter than synchrotron radiation sources alone. Their ultra-short pulses, down in the femtosecond (10-15 second) range, also allow for very brief illumination of the sample. This ensures that an image can be acquired before the specimen is destroyed by the intensity of the X-rays. This technique has enabled data collection from micrometer- to nanometer-sized crystals, and has been used to image protein nanocrystals and even larger virus particles.
However, much remains to be done to acquire images of even the smaller specimens. Small spherical viruses are among the simplest replicating systems in biology, yet they are still poorly understood. Only a very superficial picture is available today on the packing of the genetic material in intact viruses. Of particular interest are viruses that cannot be crystallized, like the human immunodeficiency virus (HIV) or the herpes simplex virus. An understanding of the functional dynamics of such viruses could shed light on key steps in a viral infection.
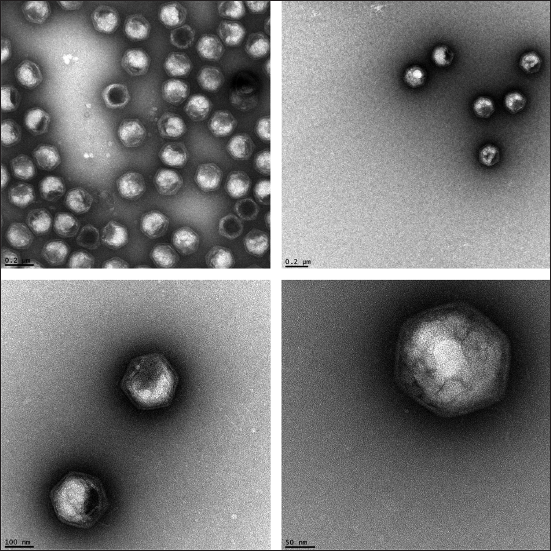
This figure shows some transmission electron microscope micrographs of the Paramecium bursarium chlorella virus. Though these display many interesting features, improvements are expected using free electron laser imaging.
(Images courtesy of Elisabeth Hartmann and Thomas Barends, MPI Heidelberg)
For the future, physicists are working toward time-resolved studies of molecular structure, function, and dynamics using X-ray lasers. This could be applied to view the folding of proteins and nucleic acids or the assembly and disassembly of biomolecular complexes. Imaging of this type could allow for the in-situ production of movies that demonstrate how molecular bonds are broken or formed. Such movies would be produced by recording billions of three-dimensional snap-shots every few femtoseconds, and then reconstructing the actions occurring at the atomic level.
Molecular-scale movies could even one day provide images of virus dynamics, which could allow scientists to develop innovative drugs and treatments. Despite the challenges associated with the imaging of such remarkably small specimens, the future of X-ray imaging techniques is indeed very bright.
Leave a Reply