Evidence that the economy is in conflict with Earth’s natural systems can be seen in the daily news reports of collapsing fisheries, shrinking forests, eroding soils, deteriorating rangelands, expanding deserts, rising carbon dioxide levels, falling water tables, rising temperatures, more destructive storms, melting glaciers, rising sea level, dying coral reefs, and disappearing species. These trends, which mark an increasingly stressed relationship between the economy and the Earth’s ecosystem, are taking a growing economic toll. At some point, this could overwhelm the worldwide forces of progress, leading to economic decline. The challenge for our generation is to reverse these trends before environmental deterioration leads to long‐term economic decline, as it did for so many earlier civilizations.
What Is a Sustainable Economy?
An environmentally sustainable economy – an eco‐economy – requires that the principles of ecology be observed in establishing the framework for formulating economic policy and that economists understand that all economic activity, indeed all life, depends on the Earth’s ecosystems – the complex of individual species living together, interacting with each other, and with their physical habitat. Millions of plant and animal species exist in an intricate balance, woven together along the food chain by nutrient cycles, the hydrological cycle, and global climate systems. Economists know how to translate goals into policy. Economists and ecologists, along with engineers, scientists, and policy makers, working together are being challenged to design and build an eco‐economy, one that can sustain progress (Brown 2001a, b; Western Governors’ Association, 3 December 2004).
Through industrial ecology, eco‐industrial parks, eco‐efficiency, Zero Discharge manufacturing, and engineering new products from agricultural materials (biorenewable resources), an eco‐economy that facilitates sustainable economic progress offer the potential for the achievements, over the long‐term, of a sustainable economy and a healthy planet. Humankind has gone outside the biotic environment for the majority of its material needs only recently. Plant‐based resources were the predominant source of energy, organic chemicals, and fibers in the West as recently as 150 years ago, and they continue to play important roles in many developing countries.
The transition to nonbiological (or nonrenewable) sources of energy and materials was relatively swift and recent in the history of the world. Biorenewable resources are by definition sustainable natural resources; that is, they are self‐renewing at a rate that ensures their availability for use by future generations. Biorenewable resources can be converted into either bioenergy or biobased engineering products. Bioenergy, also known as biomass energy, is the result of the conversion of the chemical energy of a biorenewable resource into heat and stationary power. Biobased products include transportation fuels, chemicals, and natural fibers derived from biorenewable resources. Transportation fuels are generally liquid fuels, such as ethanol or biodiesel, but compressed hydrogen and methane have also been proposed and evaluated for use in vehicular propulsion.
Chemicals may include pharmaceuticals, nutraceuticals, and other fine chemicals, but the emphasis here is on commodity chemicals, which produce high demands for biorenewable resources. An example is polylactic acid, which is derived from the fermentation of sugars hydrolyzed from cornstarch and can be converted into biobased polymers used in a variety of consumer products such as carpets.
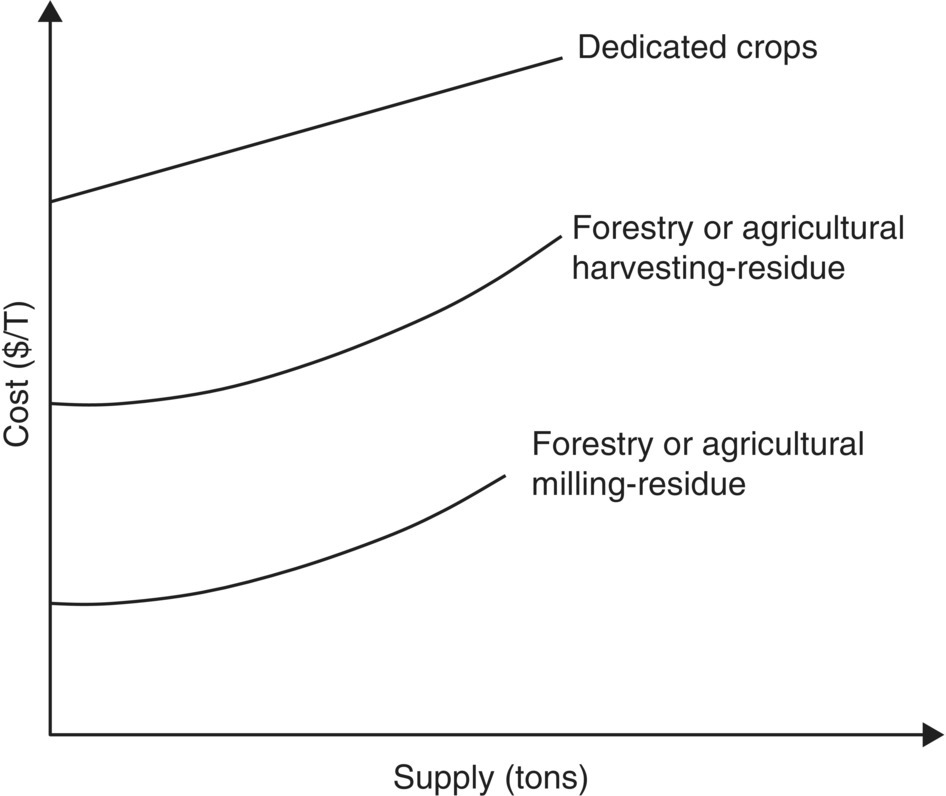
The cost of a biorenewable resource is related to the demand for the resource by a supply curve. Figure 7.9 is a generalized representation of a supply curve for the three kinds of biorenewable resources. The cost of biorenewable resources is highly variable and dependent on local conditions of supply and demand. This is particularly true for the costs involved in processing residue and wastes. Table 7.13 provides an estimate of the availability and cost of several kinds of residues and wastes along with a comparison of the costs of a few crop. The cultivation and harvesting of dedicated energy crops, on the other hand, is amenable to standardized cost estimating since information on “unit operation,” such as planting, fertilizing, and harvesting, can be readily obtained from knowledgeable sources (Brown 2001b).
Leave a Reply