This concept of antimatter doesn’t just apply to electrons. For every type of fundamental particle in the universe there exists a corresponding type of antiparticle, at least in principle. The antiparticle has the same mass and spin as the particle partner, but opposite electric charge. (The uncharged, massless particle called the photon is one important exception.) In addition to positrons, there are antiprotons and antineutrons, for example.
There is a serious imbalance in the number of particles we actually observe compared to the number of antiparticles. For some reason, it turns out that antiparticles are rare out in the real world compared to their normal matter counterparts. You could say that a lot of antimatter is “missing.” It is still a bit of a mystery why our universe has so much more matter than antimatter. Nevertheless, modern particle accelerators routinely create antimatter during high energy physics experiments.
Recall that mass is understood to be just another form of energy (based on Einstein’s famous equivalence, E = mc2). Energy can be converted to mass if it is concentrated enough under the right conditions. Take an electron-positron pair for example. If you can concentrate an amount of energy greater than or equal to the mass of two electrons times the speed of light squared, then an electron and a positron can spontaneously appear (while that energy disappears). This phenomenon is called “pair production,” and it can happen to any particle-antiparticle pair, not just electrons and positrons.
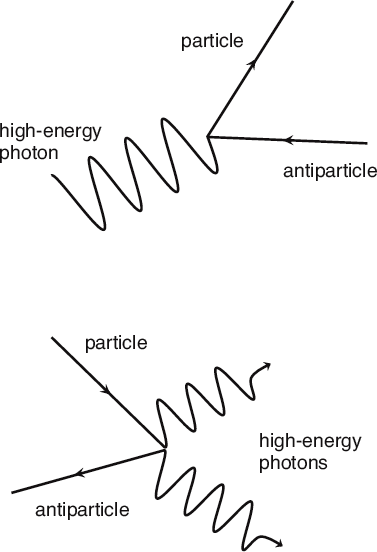
A photon with high enough energy can spontaneously convert into a particle-antiparticle pair (top). Conversely, whenever a particle collides with its antiparticle, they annihilate and leave behind a few high-energy photons (bottom).
The opposite effect is also predicted and observed. If a particle somehow meets a specimen of its antiparticle, then the two masses annihilate each other, disappearing into an equivalent amount of energy. That energy will generally appear as two or more photons.
All of the quantum mechanics would be the same as it is in our world if the whole cast of particle characters were replaced with their antiparticle partners. Positrons would be bound to nuclei made of antiprotons and antineutrons, and find themselves in stationary quantum states with all the same features we have discussed. They could form atoms of antihydrogen or any other element in the periodic table. Those antielements could even form antimolecules, because molecular binding would work exactly the same way, too. Thus two antihydrogen atoms could attach to one antioxygen atom to make an antimatter molecule that would have properties identical to our water molecules.
A few minor problems arise, however, if we want to bring a sample of this antiwater into the lab to study it. That’s because the real world laboratory is full of real electrons and other matter particles. Electrons as we have seen occupy the volume of all ordinary atoms, whether they be in air molecules, the top of the lab bench, or glass beakers. The positrons which occupy the analogous place in our antiwater molecules are positively charged, so they are strongly attracted to any nearby negatively charged electrons. As soon as they get close together, bam! They annihilate into pure energy. With the positrons gone, our antiwater molecules fall apart, and the antiprotons in their nuclei will find some regular protons in the lab, and bam! again.
Unless we somehow isolate the drop of antiwater from all other matter in the lab, it will quickly and completely disappear in a burst of energy. How much energy? Let’s say we have a very tiny drop, with a mass of only 1 gram. The energy released when it annihilates with a gram of matter will be equivalent to the explosion of about 40,000 tons of TNT—more than twice that of the atomic bombs deployed during World War II.
Leave a Reply