Today, we know that visible light isn’t the only kind of electromagnetic wave out there. The radio waves picked up by your cell phone and the microwaves that cooked your leftover meatloaf are both waves that fit into a broad electromagnetic spectrum. The only difference between these different types of waves is the rate at which they oscillate—or move back and forth—a quantity known as frequency and represented by the symbol f. According to classical physics, the electromagnetic spectrum is continuous, and every frequency along the way is as permissible as every other.
In addition to frequency, we can describe electromagnetic waves by measuring how “long” they are. Specifically, the distance from crest to crest of the wave is known as wavelength and represented by the Greek symbol λ. When speaking of visible light, red light has the longest wavelength (lowest frequency), while violet has the shortest wavelength (highest frequency).
The third and final important quantity that is needed to describe electromagnetic waves is the speed with which they travel. This so-called speed of light is represented by the symbol c. This is a constant value that never changes, no matter what type of electromagnetic wave you have. It also serves as a universal speed limit, since nothing can travel faster than c. Mathematically, the three quantities are related by the equation c = λf. Since all electromagnetic waves travel with the same speed, longer wavelengths have lower frequencies, and shorter wavelengths have higher frequencies.
Most light sources, such as the sun or an incandescent light bulb, actually emit light spanning a range of frequencies. Physicists also use special light sources that emit pure light of a single frequency, or monochromatic light.
DEFINITION
The wavelength of a wave is the distance between two successive crests.
The frequency of the wave is the number of oscillations, or movements back and forth, per unit time.
Light emitted at a single pure frequency (or wavelength) is called monochromatic.
Compared to the people-sized water waves we observed earlier during our fishing trip, light waves are remarkably short. In fact, the wavelength of the soft orange light emitted from a typical streetlight is about 60 millionths of a centimeter (0.00006 cm). This means you could fit over 15,000 of these little orange light waves across the top of a thumbtack!
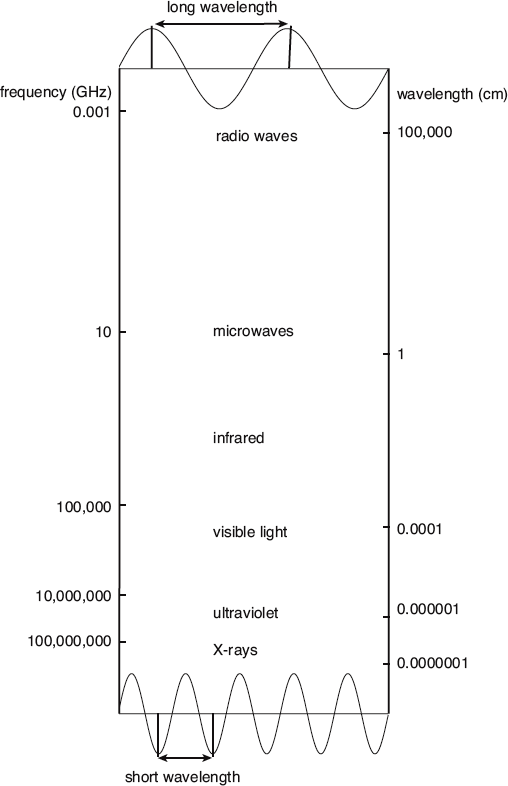
The “size” of an electromagnetic wave is determined by its wavelength or its frequency. All possible frequencies are allowed, and these span a broad spectrum. Note that one gigahertz (1 GHz) is equal to one billion hertz (1,000,000,000 Hz), where a “hertz” means one cycle per second.
It is this very “smallness” of light waves that tripped up Newton in the first place. Light waves are extremely short compared to the dimensions of your ordinary lens, mirror, or prism. This means that when light bounces off or passes through one of these objects, the deviation from straight-line motion is virtually imperceptible. For this reason, Newton’s geometric optics works just fine for almost all everyday applications. It begins to break down only when light interacts with very small objects, like Young’s thin double slits.
If we travel along the electromagnetic spectrum toward longer and longer wavelengths, we soon arrive at the region of infrared radiation. While infrared radiation is not visible to the naked eye, instruments such as night-vision goggles can easily detect it. These work by sensing the thermal radiation—essentially the heat—emitted by the objects in view.
DEFINITION
When an object or substance is heated or excited until it glows, the observed pattern of frequencies (or wavelengths) emitted is referred to as its emission spectrum.
Thermal radiation is the type of electromagnetic wave emitted by a physical object merely as a result of its temperature.
The iron fireplace poker illustrates a particularly interesting connection between heat and electromagnetism. When you lift the poker from its rack, it appears pitch black. This is because it reflects no visible light into your eye. If there are no other sources of light in the room, the only radiation you can detect from the poker is thermal radiation, and this lies in the (invisible) infrared region of the spectrum.
If you set the poker into the fire, it will begin to heat up. As it heats, the thermal radiation emitted by the poker moves from the infrared region of the spectrum to the visible region. It glows red first, and if you heat it even more, it will glow white. If you could make the fire hot enough, it would even start to look blue. At every temperature along the way, the poker emits a characteristic range of colors called an emission spectrum. But the color your eye detects is more or less determined by the frequency with which the most energy is emitted. Physicists use the term intensity as a measure of how much energy is emitted at any given moment. It can also be thought of as a measure of brightness.
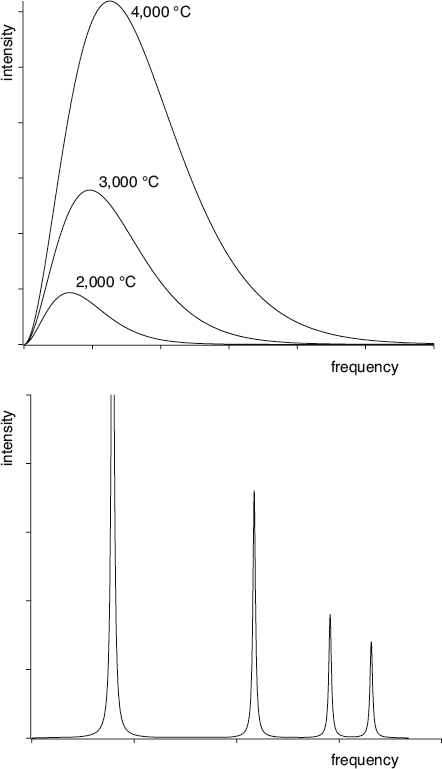
This graph displays three emission spectra for a solid object heated to three different temperatures (top), compared to the emission spectrum of an excited gas (bottom). The emission spectrum for a heated gas is essentially the same independent of its temperature (only the thickness of the lines will change).
Changing the color of a fireplace poker is not only a neat way to impress your dinner guests. It also has great scientific utility. This is because there is a universal relationship between the spectrum of a glowing object and its temperature, with very little dependence on the exact nature of the heated object. This universal relationship was discovered by the British photographer Thomas Wedgewood, who noticed that all objects heated to a certain temperature glowed with about the same color. This means, of course, you can tell how hot an object is just by the color it glows, without ever having to touch it.
QUANTUM LEAP
Thomas Wedgewood’s universal color-temperature relationship can be used to show that the sun’s spectral output peaks at a wavelength of about 0.00005 cm. Coincidentally, this is dead center within the range of light visible to the human eye! How did the sun adapt to perfectly accommodate our vision? One answer comes from none other than Wedgewood’s cousin—a man by the name of Charles Darwin—whose theories imply that the eyes of humans evolved in response to the sun rather than the other way around.
In contrast to the broad and continuous spectra emitted by heating solid materials, electrically excited gases emit light at a few very distinct frequencies—a few narrow lines, each its own pure color. Conversely, when white light is shined through a gas, the gas preferentially absorbs light at those exact same frequencies. Gases are characterized by discrete sets of spectral lines, and each type of gas has its own unique “line spectrum.” We will explore the significance of this difference between solids and gases to the development of quantum physics.
We pointed out that Maxwell’s classical electrodynamics could be used to explain nearly every electromagnetic phenomenon we observe in our daily lives. The spectra emitted by heated solids and excited gases, however, proved to be stubborn exceptions. The seemingly exceptional cases exposed some fundamental flaws that would ultimately knock classical physics from its pedestal and usher in the era of quantum physics.
Leave a Reply