Under initial conditions, one unit (1 ac-ft) of bulk reservoir rock contains
Connate water: 43,560 × φ × Swi ft3
Reservoir gas volume: 43,560 × φ × (1 – Swi) ft3
Surface units of gas: 43,560 × φ × (1 – Swi) ÷ Bgi SCF
In many reservoirs under water drive, the pressure suffers an initial decline, after which water enters the reservoir at a rate equal to the production rate and the pressure stabilizes. In this case, the stabilized pressure is the abandonment pressure. If Bga is the gas volume factor at the abandonment pressure and Sgr is the residual gas saturation, expressed as a fraction of the pore volume, after water invades the unit, then under abandonment conditions, a unit (1 ac-ft) of the reservoir rock contains
Water volume: 43,560 × φ × (1 – Sgr) ft3
Reservoir gas volume: 43,560 × φ × Sgr ft3
Surface units of gas: 43,560 × φ × Sgr ÷ Bga SCF
Unit recovery is the difference between the initial and the residual surface units of gas, or

The recovery factor expressed in a percentage of the initial gas in place is
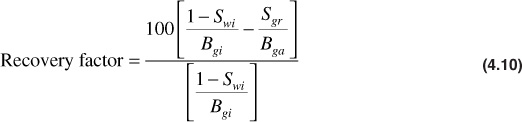
Suppose the Bell Gas Field is produced under a water drive so that the pressure stabilizes at 1500 psia. If the residual gas saturation is 24% and the gas volume factor at 1500 psia is 0.01122 ft3/SCF, then the initial unit reserve or unit recovery is
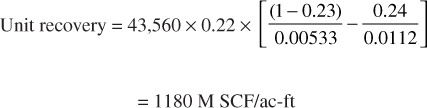
The recovery factor under these conditions is
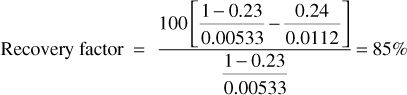
Under these particular conditions, the recovery by water drive is the same as the recovery by volumetric depletion, illustrated in section 4.3. If the water drive is very active and, as a result, there is essentially no decline in reservoir pressure, unit recovery and the recovery factor become


For the Bell Gas Field, assuming a residual gas saturation of 24%,
Unit recovery = 43,560 × 0.22 × (1 – 0.23 – 0.24) ÷ 0.00533
= 953 M SCF/ac-ft
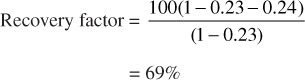
Because the residual gas saturation is independent of the pressure, the recovery will be greater for the lower stabilization pressure.
The residual gas saturation can be measured in the laboratory on representative core samples. Table 4.2 gives the residual gas saturations that were measured on core samples from a number of producing horizons and on some synthetic laboratory samples. The values, which range from 16% to 50% and average near 30%, help to explain the disappointing recoveries obtained in some water-drive reservoirs. For example, a gas reservoir with an initial water saturation of 30% and a residual gas saturation of 35% has a recovery factor of only 50% if produced under an active water drive (i.e., where the reservoir pressure stabilizes near the initial pressure). When the reservoir permeability is uniform, this recovery factor should be representative, except for a correction to allow for the efficiency of the drainage pattern and water coning or cusping. When there are well-defined continuous beds of higher and lower permeability, the water will advance more rapidly through the more permeable beds so that when a gas well is abandoned owing to excessive water production, considerable unrecovered gas remains in the less permeable beds. Because of these factors, it may be concluded that generally gas recoveries by water drive are lower than by volumetric depletion; however, the same conclusion does not apply to oil recovery, which is discussed separately. Water-drive gas reservoirs do have the advantage of maintaining higher flowing wellhead pressures and higher well rates compared with depletion gas reservoirs. This is due, of course, to the maintenance of higher reservoir pressure as a result of the water influx.
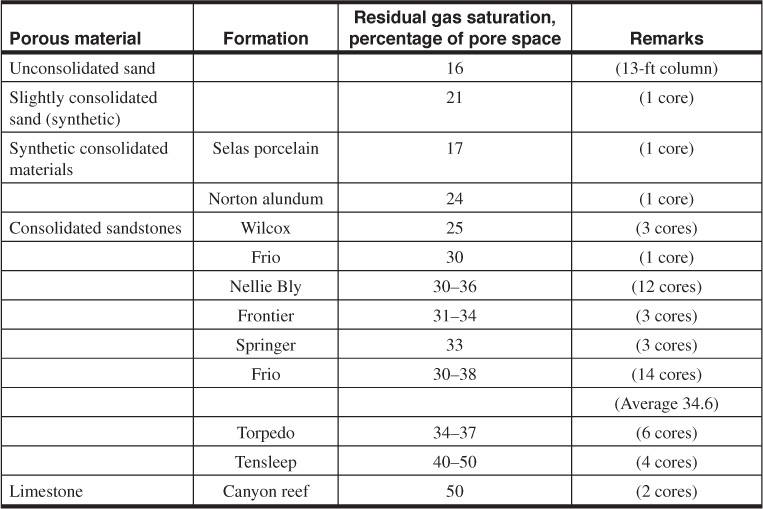
Table 4.2 Residual Gas Saturation after Waterflood as Measured on Core Plugs (after Geffen, Parish, Haynes, and Morse)5
In calculating the gas reserve of a particular lease or unit, the gas that can be recovered by the well(s) on the lease is important rather than the total recoverable gas initially underlying the lease, some of which may be recovered by adjacent wells. In volumetric reservoirs where the recoverable gas beneath each lease (well) is the same, the recoveries will be the same only if all wells are produced at the same rate. On the other hand, if wells are produced at equal rates when the gas beneath the leases (wells) varies, as from variable formation thickness, the calculated initial gas reserve of the lease, where the formation is thicker, will be less than the initial actual recoverable gas underlying the lease.
In water-driven gas reservoirs, when the pressure stabilizes near the initial reservoir pressure, the lowest well on structure will divide its initial recoverable gas with all updip wells in line with it. For example, if three wells in line along the dip are drilled at the updip edge of their units, which are presumed equal, and if they all produce at the same rate with the same producing life, then the lowest well on structure will recover approximately one-third of the gas initially underlying it. If the well is drilled further downstructure near the center of the unit, it will recover still less. If the pressure stabilizes at some pressure below the initial reservoir pressure, the recovery factor will be improved for the wells low on structure. Example 4.1 shows the calculation of the initial gas reserve of a 160-acre unit by volumetric depletion, partial water drive, and complete water drive.
Example 4.1 Calculating the Initial Gas Reserve of a 160-acre Unit of the Bell Gas Field by Volumetric Depletion and under Partial and Complete Water Drive
Given
Average porosity = 22%
Connate water = 23%
Residual gas saturation after water displacement = 34%
Bgi = 0.00533 ft3/SCF at pi = 3250 psia
Bg = 0.00667 ft3/SCF at 2500 psia
Bga = 0.03623 ft3/SCF at 500 psia
Area = 160 acres
Net productive thickness = 40 ft
Solution
Pore volume = 43,560 × 0.22 × 160 × 40 = 61.33 × 106 ft3
Initial gas in place is
G1 = 61.33 × 106 × (1 – 0.23) ÷ 0.00533 = 8860 MM SCF
Gas in place after volumetric depletion to 2500 psia is
G2 = 61.33 × 106 × (1 – 0.23) ÷ 0.00667 = 7080 MM SCF
Gas in place after volumetric depletion to 500 psia is
G3 = 61.33 × 106 × (1 – 0.23) ÷ 0.03623 = 1303 MM SCF
Gas in place after water invasion at 3250 psia is
G4 = 61.33 × 106 × 0.34 ÷ 0.00533 = 3912 MM SCF
Gas in place after water invasion at 2500 psia is
G5 = 61.33 × 106 × 0.34 ÷ 0.00667 = 3126 MM SCF
Initial reserve by depletion to 500 psia is
G1 – G3 = (8860 – 1303) × 106 = 7557 MM SCF
Initial reserve by water drive at 3250 psia is
G1 – G4 = (8860 – 3912) × 106 = 4948 MM SCF
Initial reserve by water drive at 2500 psia is
(G1 – G5) = (8860 – 3126) × 106 = 5734 MM SCF
If there is one updip well, the initial reserve by water drive at 3250 psia is

The recovery factors calculate to be 85%, 65%, and 56% for the cases of no water drive, partial water drive, and full water drive, respectively. These recoveries are fairly typical and can be explained in the following way. As water invades the reservoir, the reservoir pressure is maintained at a higher level than if water encroachment did not occur. This leads to higher abandonment pressures for water-drive reservoirs. Because the main mechanism of production in a gas reservoir is that of depletion or gas expansion, recoveries are lower, as shown in Example 4.1.
Agarwal, Al-Hussainy, and Ramey conducted a theoretical study and showed that gas recoveries increased with increasing production rates from water-drive reservoirs.6 This technique of “outrunning” the water has been attempted in the field and has been found successful. Matthes, Jackson, Schuler, and Marudiak showed that ultimate recovery increased from 69% to 74% by increasing the field production rate from 50 to 75 MM SCF/D in the Bierwang Field in West Germany.7 Lutes, Chiang, Brady, and Rossen reported an 8.5% increase in ultimate recovery, with an increased production rate in a strong water-drive Gulf Coast gas reservoir.8
A second technique used in the field is the coproduction technique discussed by Arcaro and Bassiouni.9 The coproduction technique is defined as the simultaneous production of gas and water. In the coproduction process, as downdip wells begin to be watered out, they are converted to high-rate water production wells, while the updip wells are maintained on gas production. This technique enhances the production of gas by several methods. First, the high-rate downdip water wells act as a pressure sink for the water because the water is drawn to these wells. This retards the invasion of water into productive gas zones in the reservoir, therefore prolonging useful productive life to these zones. Second, the high-rate production of water lowers the average pressure in the reservoir, allowing for more gas expansion and therefore more gas production. Third, when the average reservoir pressure is lowered, immobile gas in the water-swept portion of the reservoir could become mobile. The coproduction technique performs best before the reservoir is totally invaded by water. Arcaro and Bassiouni reported the improvement of gas production from 62% to 83% in the Louisiana Gulf Coast Eugene Island Block 305 Reservoir by using the coproduction technique instead of the conventional production approach.
Leave a Reply