Atmospheric hazards are comprised of three primary types: oxygen deficient atmospheres, explosive/flammable atmospheres, and toxic atmospheres. These types of hazards require air monitoring and ventilation before entering a manhole or other confined space. Air monitoring equipment is discussed later in this chapter. It should be noted that atmospheric hazards caused or contributed to by an industrial user discharge that result in the presence of toxic gases, vapors, or fumes within factories and workplaces in a quantity that may cause acute worker health and safety problems is violation of the specific prohibition at 40 CFR 403.5(b)(7).
Oxygen Deficient Atmosphere
The minimum OSHA requirement for oxygen concentration in the atmosphere is 19.5%. A Self‐ Contained Breathing Apparatus is necessary to enter an atmosphere with less than 19.5% oxygen. An oxygen enriched atmosphere, which exists when the oxygen concentration is greater than 25%, is also considered hazardous because of its ability to support combustion. Normal air consists of the gases listed in Table 5.7. Other gases, such as nitrogen and carbon dioxide, which are harmless under normal conditions, may build up in confined spaces in quantities large enough to displace the oxygen necessary to support life. When the concentration of oxygen in the atmosphere falls to 10–16%, a person will experience shortness of breath. Loss of consciousness will occur at a 6–10% oxygen concentration and death will occur rapidly when the concentration of oxygen falls below 6%.
Toxic Atmosphere
There are various guidelines for assessing chemical hazards in the atmosphere. The TLVs are guidelines developed and published by the American Conference of Governmental Industrial Hygienists to be used for identifying and controlling potential hazards. One form of the TLV, the TWA, refers to the vapor phase concentration a worker may be exposed to for an 8‐hour workday or 40‐hour work week without chronic or acute health effects. TLV‐TWA numbers are sometimes used to calculate industrial user discharge screening levels for VOCs. See TLVs for various chemical substances at http://www.acgih.org/tlv‐bei‐guidelines/tlv‐chemicalsubstances‐introduction.
The EPA has issued a guidance document entitled Guidance to Protect POTW Workers from Toxic and Reactive Gases and Vapors (EPA 812‐B‐92‐001) June 1992. This document should be used to evaluate the potential for exposure to toxic atmospheres and necessary steps for avoiding contact with such atmospheres. Hydrogen sulfide (H2S) is a common gas found in the collection system. The gas, which is formed by anaerobic decomposition of organic matter, is heavier than air and tends to collect at the bottom of an enclosed space. At low concentrations, hydrogen sulfide has an odor of rotten eggs, at higher concentrations, however, the olfactory system becomes impaired and the gas cannot be detected by smell. The TLV‐TWA for hydrogen sulfide is 100 ppm. At higher concentrations of H2S, damage can occur to the eyes, nervous system, and respiratory system. A caustic solution (sodium sulfide) is formed when the gas comes into contact with moist tissue, such as in the eyes and respiratory tract, which causes the irritation and danger from the chemical. At concentrations of 500–1000 ppm, the respiratory system is paralyzed and death will occur.
Table 5.7 Atmospheric constituents.
Gas | Volume (%) |
Nitrogen | 78.09 |
Oxygen | 20.95 |
Argon | 0.93 |
Carbon dioxide | 0.03 |
Hydrogen cyanide gas may be generated when cyanide salts react with an acidic wastestream. Cyanide salts are often found in plating baths and metal‐finishing facilities. The gas causes death by preventing the transfer of oxygen within the bloodstream.
Chlorine (Cl2) gas may be encountered at plating facilities where it is used for cyanide destruction. Aside from the irritating odor, chlorine gas is corrosive in the presence of moisture. It combines with moisture in the lungs and the respiratory system to form hydrochloric acid. Pulmonary edema (fluid in the lungs) may occur at 50 ppm and at 1000 ppm death occurs rapidly.
Carbon monoxide (CO) is another gas that is generated in a collection system from anaerobic decomposition of organic materials. Asphyxiation occurs from exposure to this gas because the hemoglobin of the blood has 300 times more affinity for carbon monoxide than for oxygen. Carbon monoxide combines with hemoglobin to form carboxy hemoglobin. As a result, blood cells with CO cannot transport oxygen to body tissues, and death occurs.
Toxic vapors also present a hazard to inspectors and sampling personnel. Vapors are the volatile form of substances that are normally in a solid or liquid state. Chlorinated solvents used in degreasing or photoresist developing operations generate vapors that may accumulate in the collection system. Some of the vapors have an anesthetic effect when inhaled. In addition, the vapors are generally heavier than air, causing oxygen in a confined space to be displaced which may create an oxygen deficiency in that space.
Chronic Industrial Exposure
Chronic toxicity in hazardous waste management is most often caused by long‐term, low‐level exposure to hazardous chemicals. Chronic toxicity is difficult to quantify because less is known about the long‐term effects of chemicals compared to acute toxicity evaluations. A typical dose–response curve for chronic toxicity is shown in Figure 5.16.
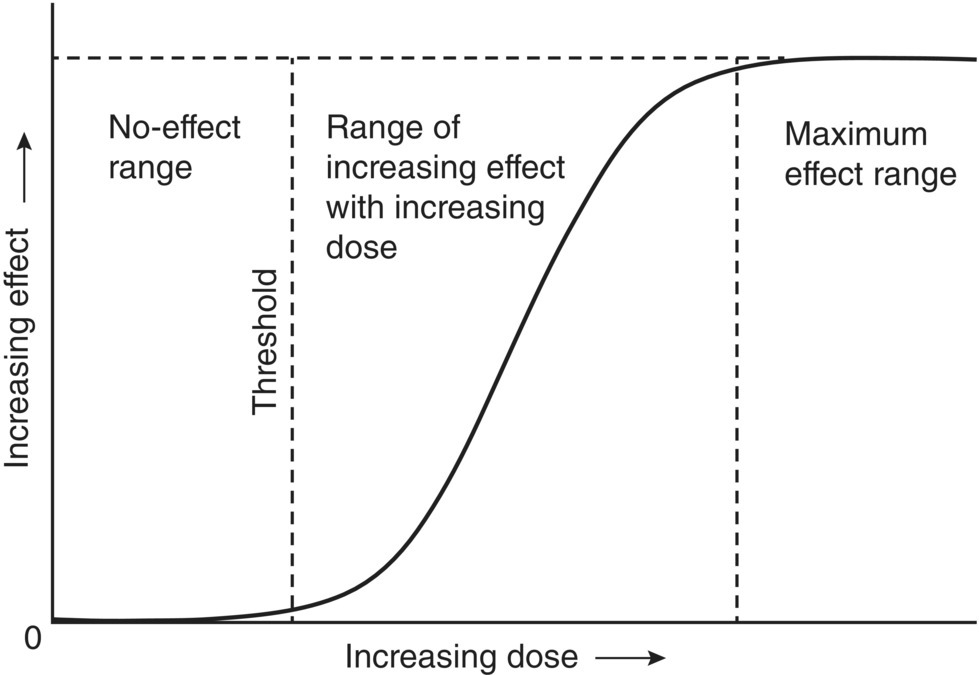
At low contaminant concentrations, essentially no evidence of toxicity is exhibited, and this is called the no‐effect region. The basis for the lack of response from zero dose to the threshold dose is a biochemical or physiological defense, such as detoxification or excretion, that prevents the effect from occurring. The middle segment rises from a point where some toxicity is shown (the threshold), and then increases in a sigmoidal fashion until a maximum effect is found. As the exposure to the chemical is increased, the detoxification mechanisms are overwhelmed and the effects are exhibited.
Accidental Chlorine Gas Release: Case Study
The Pierce County Chambers Creek Wastewater Treatment facility in Tacoma, Washington uses chlorine for disinfection; the gas is stored on site in a 1‐T container. The maximum anticipated storage inventory is ten containers (20 000 lb), which exceeds the threshold for applicability of the Risk Management Plan (RMP) requirements specified in the Accidental Release Prevention Program (USEPA 1996). The threshold for chlorine is 2500 lb. The potential off‐site consequences of an accidental release of this poisonous gas are serious, and an evaluation of the situation is called for. The methodology used for this analysis follows the approach outlined in EPA’s risk management program guidance for wastewater treatment plants (USEPA 1998b) and in the guidance provided in the White’s Handbook of chlorination and alternative disinfectants (2010).
According to the Accidental Release Prevention Program (USEPA 1996), the toxic gas worst case scenario must assume the release of the single largest vessel or container over a period of 10 minutes. The largest vessel at Chambers Creek is the 1‐T container noted earlier.
For worst‐case scenarios, releases are assumed to take place without consideration for physical cause or likelihood of occurrence. Only passive mitigation measures (e.g. dikes, enclosures) can be considered, while active mitigation measures cannot be included. Furthermore, the rule states that toxic gases should be evaluated at ambient temperatures unless the gas is liquefied by refrigeration at ambient pressure. The chlorine containers at the treatment facility can be stored either inside or outside the building. For a worst‐case scenario, it was assumed that a container was ruptured outside the building.
The release rate, calculated for the characteristics of a vapor release, was 200 lb/min for 10 minutes. An alternative release scenario, assuming a slower rate of release owing to the use of active mitigation measures, was calculated as 31.3 lb/min over 20 minutes. For the remainder of this case study, we consider both the worst‐case scenario and the alternative release possibly just described.
Leave a Reply