The development of new materials and better utilisation of existing materials has been central to the advancement of aerospace engineering. Advances in the structural performance, safety, fuel economy, speed, range and operating life of aircraft has been reliant on improvements to the airframe and engine materials. Aircraft materials have changed greatly in terms of mechanical performance, durability, functionality and quality since the first powered flight by the Wright Brothers in 1903. Furthermore, the criteria which are used to select materials for aircraft have also changed over the past 100 years. Figure 2.1 presents a timeline for the approximate years when new criteria were introduced into the selection of aircraft materials.
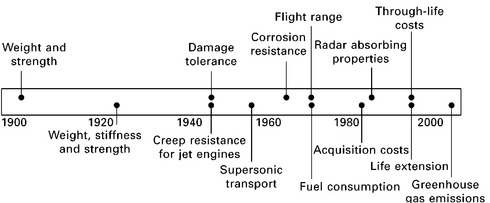
2.1 Historical timeline indicating when key criteria for materials selection were introduced into aircraft design.
The main criteria for materials selection for the earliest aircraft (c. 1903–1920) was minimum weight and maximum strength. The earliest aircraft were designed to be light and strong; other design criteria such as cost, toughness and durability were given less importance in the quest for high strength-to-weight. Many of the criteria which are now critical in the choice of materials were not recognised as important by the first generation of aircraft designers, and their goal was simply to use materials that provided high strength for little weight. At the time the best material to achieve the strength-to-weight requirement was wood.
The situation changed during the 1920s/1930s when the criteria for materials selection widened to consider a greater number of factors affecting aircraft performance and capability. The design of aircraft changed considerably as commercial and commuter aviation became more popular and the military began to recognise the tactical advantages of fast fighters and heavy bombers. Improved performance from the 1930s led to aircraft capable of flying at fast speeds over long distances while carrying heavy payloads. The requirement for high strength-to-weight remained central to the choice of material, as it had with earlier aircraft, but other criteria such as high stiffness and durability also became important. Higher stiffness allowed sleeker and more compact designs, and hence improved performance. These new criteria not only required new materials but also the development of new production methods for transforming these materials into aircraft components. Aluminium alloys processed using new heat treatments and shaped using new metal-forming processes were developed to meet the expanding number of selection criteria. The importance of availability emerged as a critical issue in the selection of materials during the Second World War. For example, the supply of aluminium to Japan was cut off in the late 1930s/early 1940s, which forced their military to use magnesium in the construction of many fighter aircraft.
Major advances in aerospace technology, particularly jet aircraft, firstgeneration helicopters and rockets/missiles, occurred shortly after World War II. These advances placed greater demands on the performance requirements of the the airframe and engine materials. Another significant milestone was the introduction of pressurised cabin aircraft for high altitude flight during the 1940s. The increased pressure loads exerted on the fuselage led to the development of stressed skin panels made using high-strength material.
Around the same time, the need for materials with fatigue and fracture properties emerged as a critical safety issue, and represents the introduction of the damage tolerance criterion. Damage tolerance is the capability of an aircraft structure to contain cracks and other damage below a critical size without catastrophic failure. The unexpected failure of aircraft structures was common before and, in some instances, during World War II. Aviation was considered a high risk industry and aircraft crashes caused by catastrophic structural failures were common. Designers attempted to minimise the risk by building bulky structures which made the aircraft heavy, but structural failures continued leading to many crashes. The fatigue of metals became more widely recognised as an important issue in the mid-1950s when two Comet airliners, the first of a new generation of civil jet airliners, crashed owing to fatigue-induced cracks in the fuselage. The Comet accidents occurred in the post-war era when civil aviation was starting to boom, and the crashes threatened public confidence in aviation safety. Fracture toughness and fatigue resistance joined other important properties such as weight, stiffness and strength as essential properties in the choice of aircraft materials.
The development of supersonic aircraft together with advances in rocket technology during the 1960s prompted the need for high-temperature materials. The aerospace industry invested heavily in the development of new materials for supersonic airliners such as Concorde, high-speed fighters and surveillance aircraft for the Cold War, and spacecraft and satellites for the Space Race. The investments led to the development of heat-resistant airframe materials such as titanium alloys and special aluminium alloys that were capable of withstanding frictional heating effects during supersonic flight without softening. The need for more powerful engines for aircraft and rockets also drove the development of high-temperature materials capable of operating above 800 °C. New types of nickel-based alloys and other heat-resistant materials were developed to survive within the hottest sections of jet engines.
The need for damage-tolerant materials became more intense in the late 1970s when unexpected failures occurred in ultra-high-strength steel components in United States Air Force (USAF) aircraft. It became clear that the failures involved manufacturing defects and fatigue cracks so small that they could not be found reliably. The USAF introduced a damage tolerant design philosophy which accepted the presence of cracks in aircraft and managed this by achieving an acceptable life by a combination of design and inspection. Achieiving this required the use of materials that were resistant to fatigue cracking and failure.
The certification of new commercial aircraft required manufacturers to demonstrate that fatigue cracks could be detected before reaching the critical length associated with catastrophic failure. Aviation safety authorities such as the FAA introduced stringent regulations on the damage tolerance of safety-critical structures. New commercial aircraft would not be certified and permitted to fly unless new criteria on damage tolerance were met. This change in the certification requirements further increased the need for damage-tolerant materials with excellent fracture toughness and fatigue properties for both airframe and engine applications.
Although always important, weight reduction of civil aircraft became critical during the 1970s owing to rising fuel costs and the revenue opportunities associated with increased range and heavier payload. The OPEC fuel crisis of the 1970s, when the price of Avgas jumped by more than 500%, threatened the financial viability of the global aviation industry and sent many airline companies broke. The aerospace industry implemented new measures to minimise weight and maximise structural performance, and this included the greater use of higher-strength aluminium alloys and the introduction of carbon-epoxy fibre composite materials into secondary structures such as engine cowlings and undercarriage doors.
The realisation of the financial benefits of extending the life of ageing aircraft during the 1980s and 1990s provided greater focus on improved damage tolerance and corrosion resistance. With the rising cost of new aircraft and greater competition among airline companies, including the introduction of low-cost carriers into the aviation market, the need to prolong the operating life of aircraft became critical. New aluminium alloys with improved corrosion resistance and composites materials which are completely resistant to corrosion were used in greater quantities. The 1990s was an era when factors such as the costs of manufacturing and maintenance became increasingly important in the choice of materials. The 1990s was also an era when novel structural materials with radar absorbing properties and low thermal emissions were used in large quantities on stealth military aircraft. Although aircraft with limited stealth capability had been in operation since the 1970s, the need for extremely low radar visibility became a critical requirement that drove the development of radar-absorbing materials.
All of the factors for materials selection outlined above apply today in the choice of materials for modern aircraft: weight, stiffness, strength, damage tolerance, fracture toughness, fatigue, corrosion resistance, heat resistance and so on. The first decade of the 21st century is characterised by an emphasis on materials that reduce the manufacturing cost (by cheaper processing and assembly using fewer parts) and lower through-life operating cost (through longer life with fewer inspections and less maintenance). Reductions in greenhouse gas emissions by reducing aircraft weight and improving engine fuel efficiency are also contemporary issues in materials selection. There is also growing interest in producing materials with environmentally friendly manufacturing processes and using sustainable materials that are easily recycled.
The evolution of aircraft technology and the associated drivers in materials selection has meant that the airframe and engine materials are constantly changing. The approximate year of introduction of the main aerospace materials is shown in Fig. 2.2. Many materials have been introduced, with most being developed specifically for aerospace but later finding applications in other sectors such as rail, automotive or engineering infrastructure. It is important to recognise that continuous improvements have occurred with each type of material since their introduction into aircraft. For example, on-going developments in aluminium alloys have occurred since the 1920s to improve properties such as strength, toughness and corrosion resistance. Similarly, advances with composite materials since the 1970s have reduced costs while increasing mechanical properties and impact toughness. Aircraft designers now have the choice of dozens of aluminium alloys with properties tailored to specific applications and operating conditions.
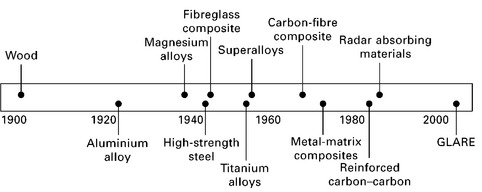
2.2 Historical timeline indicating the approximate year when the main types of materials were first used in aircraft.
We study the historical development of the major types of aerospace materials: wood, aluminium, magnesium, titanium, nickel superalloys and composites. The introduction of these materials into aircraft structures or engines, and how their usage and properties have changed over time is discussed. Also, the current status and future growth in aircraft production and how this may impact on the use of materials is examined. The on-going advances in materials technology for next-generation aircraft, helicopters and space-craft are also reviewed.
Leave a Reply