Star Trek introduced us to the fantastic notion of teleporting humans from one place to another, such as from the Starship Enterprise to planets beneath. Doing so was quite convenient, and eliminated the need for expensive landings and takeoffs. It certainly would make life more convenient if we could avoid the morning commute and just teleport to the work. But is there any fact behind this science fiction?
Two basic ingredients seem essential for teleportation. First, you need to be able to scan the outgoing object in utmost detail, so that you know its exact composition at the atomic level. This information would be required to assemble the incoming object without introducing mistakes. Second, it would be nice to have instantaneous transfer of the outgoing to the incoming object. Otherwise, teleportation across great distances (like to the next galaxy) would still take a prohibitively long time.
At first glance, quantum physics seems to rule out the former. We’ve already studied how the Heisenberg uncertainty principle places a fundamental limitation about how much you can know about a system at the atomic level. Special relativity seems to rule out the latter, since it tells us that nothing can travel faster than the speed of light. Once again, however, entangled states may help us achieve the seemingly impossible.
In 1993, a team of scientists at IBM demonstrated that, in theory, the use of entangled states could get us around the uncertainty limit. They developed an algorithm that would allow you to teleport a particle across space to another location, provided only that the initial particle was “destroyed” during the process. Also information can travel instantaneously from one particle of an entangled pair to another (Einstein’s so-called “spooky action at a distance”). So, teleportation may be real after all, thanks to quantum entanglement.
QUANTUM LEAP
Quantum teleportation is analogous to sending a fax, in that only information is transmitted and the final product relies on some sort of “feedstock” being present in the new location. One reason that we can pull this off is the indistinguishability of quantum particles, which we discussed. This guarantees the possibility of a pristine feedstock for the new object. All we need to complete the job is to transfer information about the quantum state.
So how does it work? Let’s return to our friends Alice and Bob, and examine how they might teleport a photon in Alice’s house over some distance to Bob’s. First things first, let’s take the information about the quantum state of the photon she wishes to teleport and package it into the form of a qubit, which we will label Ψt.
Next, to initiate the teleportation, Alice and Bob need to create an entangled state between two new photons. The result is a double qubit. Afterward, they separate the photons. We will refer to the one Alice takes as Ψa(b)and the one that Bob takes as Ψb(a). The letter in parentheses just indicates the identity of the entangled partner. Also, since the entangled state is a double qubit, the particle pair can exist in four potential states simultaneously.
Now, while Bob waits patiently, Alice brings her two qubits (Ψa(b)and Ψt) together to form a new double qubit we will call Ψa(t). Again, as a double qubit, it can exist in four possible states. Alice then measures the state of her double qubit. This immediately collapses its wave function, so she knows exactly which of the four entangled qubit states it is now in. Incidentally, this is the moment where Alice’s two qubits become entangled, and also the stage where the original photon, Ψt, is “destroyed.”
Because one of the two qubits in Alice’s double qubit was initially entangled with Bob’s qubit, by performing her measurement she also collapses Bob’s qubit into state Ψb(t)! She then transmits a message to Bob, using a pair of plain old classical bits (00, 01, 10, or 11), signaling which of the entangled, four-qubit states she found during her measurement.
All that is left now is for Bob to apply some straightforward operation to transform his qubit Ψb(t) into the qubit state (Ψt) that Alice specified in her classical communication. The result of this operation is that Bob’s photon is henceforth in the same quantum state as the original photon Alice wanted to transport. The photon itself wasn’t teleported, but all the information he needed to prepare an exact replica was.
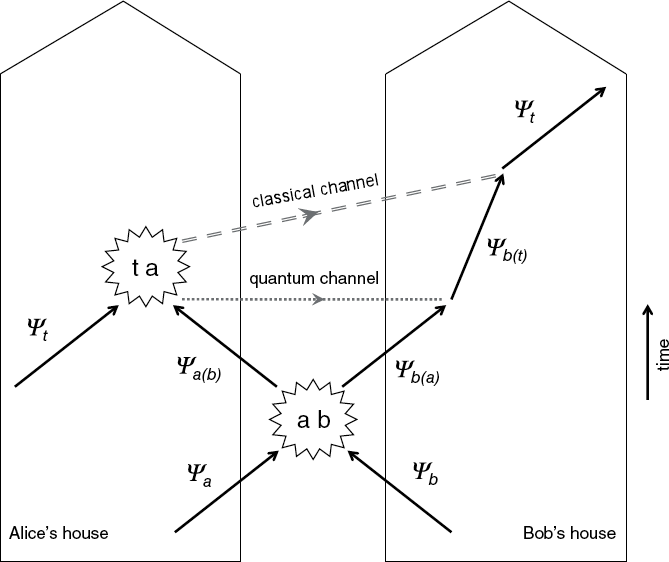
This figure illustrates how Alice and Bob could manage to teleport the photon Ψt from Alice’s house to Bob’s through the use of an entangled pair of photons originating from Ψa and Ψb. It requires two ordinary bits to be transferred via a classical channel, and information exchange between the entangled pair via a quantum channel. Here, time progresses in the upward direction.
We now see that entanglement did indeed get us around Heisenberg uncertainty. But the need to use a classical channel to transmit a few normal bits of information means that teleportation in this sense will be forever bounded by the speed of light. It appears that instantaneous teleportation will remain impossible, at least for now.
The field of quantum teleportation is still young, but it has made some steady advances. Experiments so far have demonstrated the teleportation of quantum states between photons and between ions. Molecules are the likely next step. But what about you and us? Given that we are made of lots and lots and lots of atoms (1028 or so), it’s going to take a while before we’re doing much beaming around ourselves. Moreover, in the real world, the transmission of the entangled particles can be challenging due to noise in transmission lines that could destroy the quantum correlations necessary for the teleportation to succeed.
Nevertheless, quantum teleportation is no longer mere science fiction. Although much needs to be investigated before we are teleporting everyday objects, teleportation at much smaller scales will likely find real applications. For example, it could facilitate the scaling up of quantum computers, enable a new form of long-distance quantum communication, or even pave the way to an ultra-fast, ultra-secure “quantum internet.”
Where Next?
We introduced you to a few ways that quantum physics is making our lives better. Just about any technology can be traced back to a scientific discovery. Given the breathtaking nature of some discoveries in quantum physics, it should be no surprise that it has managed to bring the seemingly impossible within our reach.
Now into its second century, quantum physics is considered to be a mature field. But the quantum realm is so rich that it’s surely far from completely understood. It’s a safe bet that quantum physics still has a few more surprises up its sleeve. And, it’s an equally safe bet that persistent quantum physicists will one day find useful things to do with them.
From page one of this book, we’ve introduced you to the main ideas of quantum physics and tried to put these into ordinary, everyday terms. We don’t expect that you’ve understood every twist and turn along the way. After all, even the quantum masters like Bohr and Einstein conceded that some quantum mysteries were bound to remain forever so. We do hope, though, that terms like duality, quantization, and entanglement no longer sound like pure gobbledygook. More important, we hope that we’ve increased your appetite to learn and to understand even more.
For our part, we are eagerly awaiting the next unexpected discovery in quantum physics. The next time quantum physics makes the headlines, which it will undoubtedly do, we hope that you’ll appreciate it just as much as we will. May the magic of quantum physics continue to explain, amaze, and inspire.
Leave a Reply