The laser is one of the best examples of a quantum application because it is so widely used. We explained that excited atoms emit photons by making a quantum jump to a lower energy state. In most cases this occurs without any influence from the outside, and emissions of this variety are called spontaneous. It turns out that this is only half the story, though, as atoms can also be prompted to emit photons through a process known as stimulated emission.
DEFINITION
Spontaneous emission of a photon results during a quantum jump that is unprovoked by external factors.
Stimulated emission of a photon results when an identical, external photon provokes the excited atom to make a quantum jump.
Stimulated emission is a fundamental physical process that was first predicted by Einstein in 1917. The importance of this process to the laser is emphasized by the origin of its name. Before it became so widely used, “laser” was just a mundane acronym for “light amplification by the stimulated emission of radiation.”
The first successful laser was built by the American physicist Theodore Maiman in 1958, and it stood on the shoulders of a similar effect, “microwave amplification by the stimulated emission of radiation.” The pithy name given to that was the maser, and its realization in 1954 was rewarded with the 1964 Nobel Prize. It was shared by American physicists Charles Townes and his Russian counterparts Nicolay Gennadiyevich Basov and Aleksandr Prokhorov.
Clearly, stimulated emission is the key common ingredient, but what exactly does this mean? The stimulated emission of a photon occurs when an excited atom is struck by a photon of the exact same frequency. In other words, one photon stimulates the emission of an identical photon. What’s more, the stimulated photon has not only the same frequency as the incident photon, it also emerges in the exact same direction and phase. (By “phase,” we simply mean that the peaks and troughs of the two corresponding electromagnetic waves occur at the exact same time. Photons with the same phase are said to be “coherent.”)
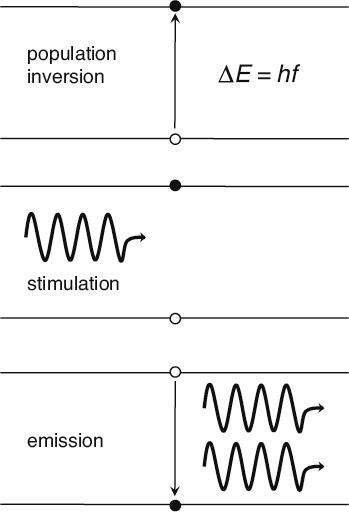
Three steps are required to produce laser light: population inversion, stimulation, and emission. Here, the horizontal lines represent energy levels, while the black dot indicates which of these the jumping electron occupies during each step.
Stimulated emission therefore requires an incoming photon (say, with frequency f) and an excited atom, and the energy of the excited state must be exactly ΔE = hf above the ground state. Otherwise, the atom has no way of making a quantum jump and emitting a photon at exactly the same frequency.
Now imagine that you have a large collection of atoms, and that many of these are in an excited state ΔE = hf above the ground state. If you introduce a photon at frequency f, it can stimulate a quantum jump in one of the excited atoms such that you end up with two identical photons. Each of these two photons can then go forth and stimulate two more identical photons, resulting in four “cloned” photons. If you have a very large population of excited atoms, you will end up with a very large army of identical, cloned photons because of the cascading effect. This is precisely the “amplification” of stimulated emission from which the laser derives its name.
Our description of amplification relies on having many excited atoms. But, as you know, atoms prefer to be in their ground state. So, something must be done to prepare a large collection of excited state atoms in the first place. This is called a population inversion, and there are various ways for achieving it. In all cases, though, you must have more atoms in the excited state than in the ground state. Otherwise, more photons will be absorbed than emitted, and your cascade will eventually peter out.
DEFINITION
A population inversion refers to the excitation of multiple atoms into a specified excited state. The inversion is complete only when more than half of the population of atoms has been excited.
The process can be aided by placing a pair of mirrors at each end of your collection of atoms. Ideally, one mirror should be 100 percent reflective while the other is partially transparent. This will allow a coherent, unidirectional beam of light to escape from one end and go on to do useful things.
The world’s first laser consisted of a flash lamp coiled around a ruby rod, which was sandwiched between a pair of mirrors. The flashing lamp created a population inversion of atoms in the ruby crystal, and the mirrors bounced photons back and forth to build up a cascade of stimulated photons. Today, lasers come in all shapes, sizes, and forms. The population of atoms, or “laser medium,” is typically some form of gas or solid. Also, different lasers use different population inversion schemes. Lasers can also be set up to emit light continuously or in pulses, and the pulse energy and pulse duration can vary greatly.
QUANTUM QUOTE
Research cannot always go directly toward the goals; you sometimes have to explore and hope something will come of it.
—Laser pioneer Arthur Schawlow
Whether you’re aware of it or not, you encounter lasers of many varieties every day. At the checkout lane in the supermarket, lasers are often used to figure out the price of the purchased items by scanning the laser beam across the bar code. The price of the item is obtained by a detector that measures the laser light reflected back from the bar code. CD and DVD players scan the surface of disks, into which little pits were burned to digitally encode the images and music we’re trying to see and hear. Of course, lasers are also used by laser printers, which use them to transfer toner onto printed pages. Chances are also good that you send print commands using a mouse that employs a laser to convert motions on your desk into movement on your display.
Lasers have also revolutionized medicine. Small, pulsed lasers are routinely used in surgery because they can deliver power very precisely to very small locations, thus preventing unnecessary damage to nearby organs or tissues. They are also very effective and minimally invasive in eye surgeries, including retinal reattachment, glaucoma treatment, and vision correction. The coherence and unidirectionality of laser beams also makes them ideal tools for precisely measuring both small and large distances. For example, laser rangefinders have been used to determine the distance between the moon and Earth to within 10 centimeters!
In 2009, advances in particle accelerator technology led to the world’s first ultra-intense and ultra-short X-ray laser. It enjoys many properties similar to tabletop lasers, but has many new features to offer. This laser uses a linear accelerator to produce an intense beam of electrons moving close to the speed of light. Electrons are sent through oscillation stages (called “undulators” or “wigglers”), where they emit X-rays. Since the electrons can’t get out of the way of the X-ray photons they have emitted, they interact together, which leads to cloned X-ray photons through the process of stimulated emission. This type of laser has been used to study molecular bonds, high temperature superconductors, the origin of magnetism, and even what might happen inside giant planets such as Jupiter.
Leave a Reply