Quantum electrodynamics is the name of the quantum field theory that applies to the electromagnetic interaction. As with classical electrodynamics, it only applies to charged particles like protons and electrons. In QED the interaction between, say, two electrons is modeled as the exchange of a fruitcake-like boson—in this case the “virtual” photon (ɣ). We saw that photons behave like discrete particles that actually carry momentum, even though they don’t have any mass (our “heavy” fruitcake analogy aside). We also know that photons have a spin of one, so they are clearly in the boson family.
We used the important caveat “virtual” because these photons cannot be directly detected. Due to the uncertainty principle, an electron is allowed to spontaneously emit a photon at any time, as long as the photon disappears again after a short enough time. Recall that it is the product of an energy fluctuation (ΔE) and its duration (Δt) that has to be sufficiently small (less than Planck’s constant or thereabouts).
Normally, the virtual photon is reabsorbed by the same electron that emitted it. But, if there is another electron nearby, a virtual photon can actually be emitted by the first electron and absorbed by the second, so long as the violation of energy conservation cannot be verified because of Heisenberg’s uncertainty principle.
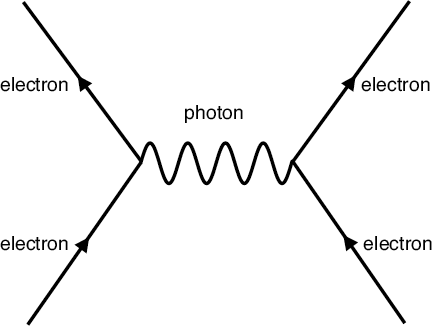
This is the Feynman diagram that shows how a single photon mediates the electromagnetic force between two electrons.
This virtual photon carries momentum with it, thus altering the paths of both the emitting and absorbing electrons. In reality, there will be a continuous exchange of a large number of virtual photons, which diverts the two paths exactly as we’d expect from the electromagnetic force. Scientists often use simplified diagrams to represent such interactions, called Feynman diagrams, after their inventor Richard Feynman. When a single photon exchange is shown in such a diagram, it actually represents the total effect of all the many virtual photons exchanged between the particles when they interact over a certain period of time.
The picture we have described here is schematic, and we have omitted many details required for the full implementation of QED. For one thing, our picture is fine for describing the repulsive force between particles with the same sign of their charge, but what about charges with opposite signs? We know they attract each other, how can an exchange of photons draw particles together? Without going into details, the best answer to this is that within the world of virtual photons, there is room for photons that carry negative momentum. If you could throw a fruitcake with negative momentum, you would begin to move in the direction of the fruitcake’s motion instead of the opposite direction.
QED has proven to be one of the most accurate theoretical models ever applied to measurements of fundamental particles. By its very nature, it is consistent with quantum physics and special relativity. It works for attraction and repulsion between particles. It even handles antiparticles just as easily as ordinary matter particles. It was the very success of this theory that inspired scientists to try to adapt its basic ideas to the other fundamental interactions. And as we’ll explain, it turns out to have worked beautifully.
Leave a Reply