What the heck is going on here? These findings were in stark contradiction to classical prediction, and they puzzled physicists for many years. The world had to wait for the one-and-only Albert Einstein to solve the problem. In a 1905 article that was nothing short of luminary, he proposed an elegant solution that explained everything, and a whole lot more.
He began by introducing something he called a “heuristic point of view”—in other words, an informed guess. Just for the sake of argument, he supposed that a light ray was not spread continuously through space in the form of a wave. Rather, he suggested that it “consists of a finite number of energy quanta which are localized at points in space, which move without dividing, and which can only be produced and absorbed as complete units.” In other words, light was composed of small, indivisible bundles that would eventually be called photons.

DEFINITION
The photon is the fundamental quantum of electromagnetic radiation, and can be thought of as a compact, localized bundle of light having a well-defined energy.
Einstein hypothesized that the energy of a given light quanta was directly proportional to the light’s frequency multiplied by Planck’s constant: E = hf. Furthermore, if an electron absorbed a photon, all of the photon’s energy was necessarily transferred to the electron. If these postulates held, he asked, what would they imply for the photoelectric effect? Let’s return to the laboratory and see.
First, consider the observation that there is a certain cut-off frequency below which no electrons are emitted. This fits nicely with Einstein’s hypotheses. The cut-off frequency must be the frequency at which the photon has just enough energy to liberate the electron in the first place. At any lower frequency, the photon doesn’t have the oomph to knock the electron free. At higher frequencies it knocks the electron free and then deposits the rest as a boost of kinetic energy.
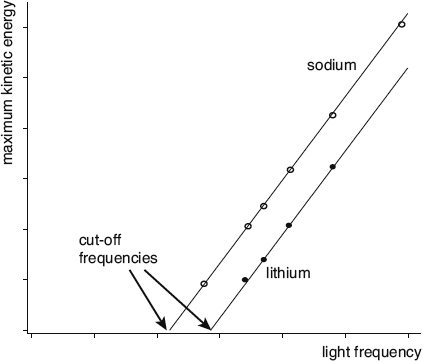
In this graphical representation of the photoelectric effect, we have plotted the maximum kinetic energy at which electrons are emitted from sodium and lithium metals as a function of the light frequency applied. The lines through the data represent Einstein’s theoretical prediction.
Second, we turn to the finding that the kinetic energy of a liberated electron is independent of the light’s intensity. According to Einstein’s point of view, as you increase the intensity of the light, you are merely increasing the number of photons, not their energy. If the frequency of the light is above the cut-off frequency, each of the streaming photons will have enough energy to liberate an electron. And since each photon has the exact same amount of energy, each will transfer the exact same amount of kinetic energy to electron it ejects. If you increase the intensity, you will have more photons and therefore more electrons at that specific kinetic energy. Einstein’s hypotheses are again consistent with the observed effects.
ATOM TRAP
Albert Einstein is most often associated with the theory of relativity. His Nobel Prize, however, was awarded for his theoretical interpretation of the photoelectric effect.
Finally, what about the question of a time delay for low intensity beams? Einstein would argue that even the weakest possible beam would have at least a few photons. So long as its frequency was above the cut-off, even one single photon would have enough energy to liberate an electron. There’d be no need for the electron to save up energy, so there would no time lag—exactly as physicists observed.
Leave a Reply